Study Reveals Details of How the Coronavirus Spike Protein Persists on Common Surfaces
Understanding chemical and structural details for how SARS-CoV-2 or other coronaviruses persist in the indoor environment is critical to control future viral spread
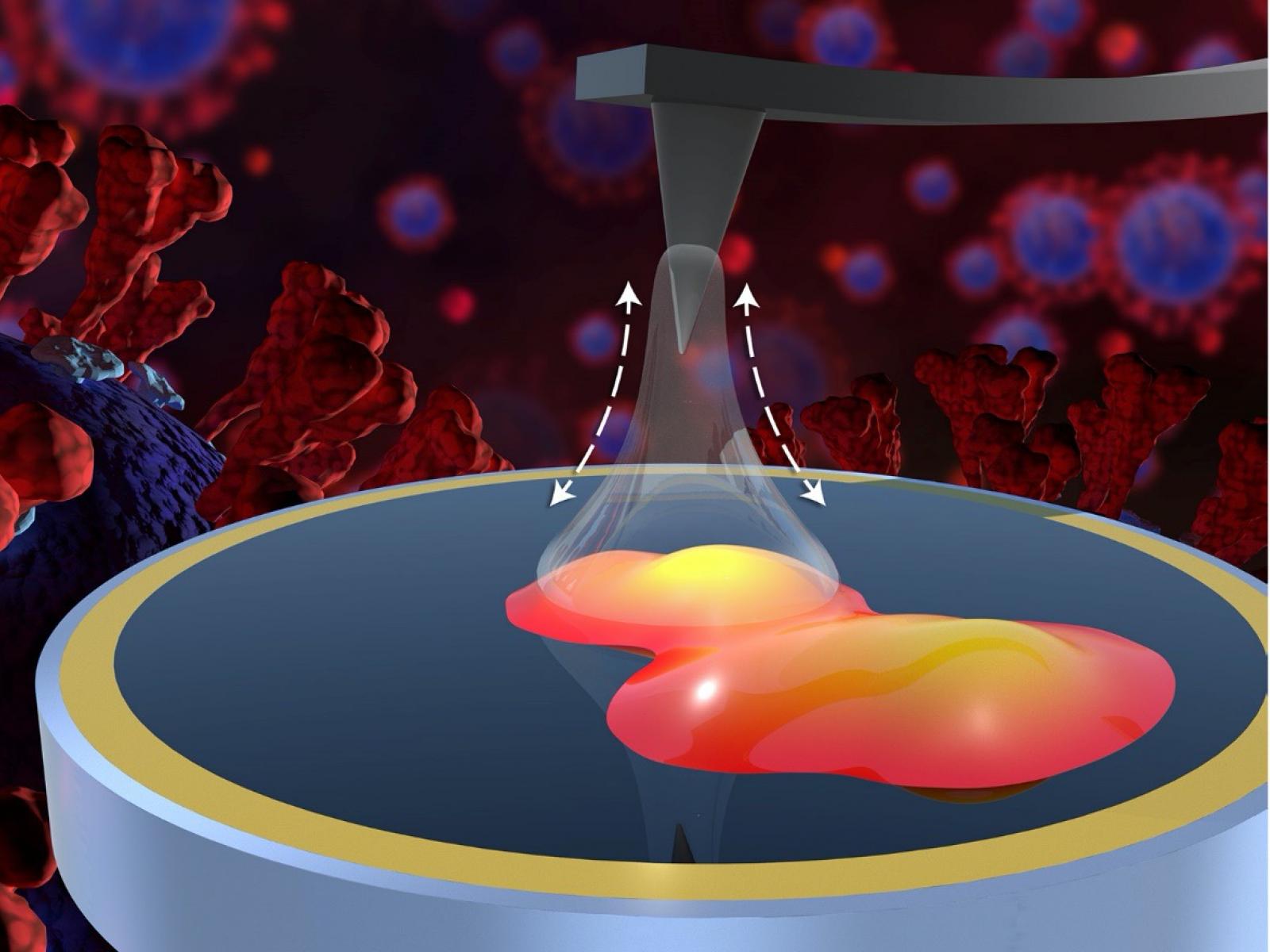
A multi-institutional team has obtained information about nanoscale interactions between the spike protein of the novel coronavirus SARS-CoV-2 and common household inorganic surfaces.
(Illustration by Michael Perkins | Pacific Northwest National Laboratory)
The Science
The novel coronavirus SARS-CoV-2 is wrapped in a lipid membrane with spike proteins pointing outward. These glycoproteins help the virus attach to a variety of surfaces found in the built environment that thereby increase the possibility of viral transmission. A multi-institutional team has measured how the SARS-CoV-2 spike protein binds to common metal oxides found in workplaces and households, obtaining structural and chemical information about nanoscale interactions between the viral protein and metal surfaces.
The Impact
The novel coronavirus spreads when people inhale virus particles in the air. However, virus particles that settle on surfaces may further viral spread. This research is the first to describe detailed mechanisms for how the SARS-CoV-2 spike protein interacts with various inorganic surfaces. Understanding how SARS-CoV-2 or other coronaviruses persist in the indoor environment is critical to control future viral spread.
Summary
The team measured adhesion forces and SARS-CoV-2 spike glycoprotein bound to common inorganic surfaces using atomic force microscopy (AFM). They coated AFM tips with metallic gold or inorganic oxides such as copper, iron, aluminum, silica, and ceria. These materials were selected because they are common in indoor environments, have diverse chemical and physical properties, and have shown a specific affinity for spike protein in previous studies.
Before the adhesion measurements, the team used a complementary novel technique, scattering-type scanning near-field optical microscopy (s-SNOM), to chemically identify and isolate the exact locations of proteins on the surface. This essential step ensured the accuracy of the measurements made using AFM.
The researchers found that the spike glycoprotein adhesion to metallic gold is about ten times stronger than all the investigated materials because gold has the highest affinity for water, and likely experiences a stronger capillary force between the protein and the native water layer on the AFM tip. However, spike protein adhesion to other materials, such as Al, Si, Fe, and CeO2, is non-specific to the properties of metal oxide surfaces as they are covered by a thin water film. The researchers concluded from this observation that the water capillary force is the dominant interaction between the spike glycoprotein and the inorganic surface.
These findings could help inform the disinfection of surfaces after exposure and the development of new engineered controls, such as air filters and surface coatings, to slow the spread of SARS-CoV-2 and other coronaviruses.
This work used microscopy and spectroscopy capabilities at EMSL, the Environmental Molecular Sciences Laboratory, a Department of Energy (DOE) Office of Science user facility located at Pacific Northwest National Laboratory.
PNNL Contact
Odeta Oafoku, odeta.qafoku@pnnl.gov, Pacific Northwest National Laboratory
Funding
The DOE Office of Science supported this research through the National Virtual Biotechnology Laboratory, a consortium of DOE national laboratories focused on the response to COVID-19, with funding provided by the Coronavirus CARES Act. The research was performed using capabilities at EMSL, a DOE Office of Science user facility sponsored by the Biological and Environmental Research program.
Published: December 10, 2021
B. O’Callahan, O. Qafoku, Waters K. et al., “Atomic force microscopy and infrared nanospectroscopy of COVID-19 spike protein for quantification of adhesion to common surfaces.” Langmuir 37, 12089-12097 (2021). [DOI: 10.1021/acs.langmuir.1c01910]