Quantum Information Science
What is quantum information science?
At the intersection between quantum mechanics and computer and information science lies at quantum information science (QIS). QIS seeks to understand how information is processed and transmitted using quantum mechanical principles. It is the merger of quantum mechanics and information and computation theory. QIS comprises four major areas: quantum computing, quantum communication, quantum sensing, and quantum foundational science.
Rather than using bits with a 1 or 0 value, like classical computers, quantum computers work by processing information in the form of qubits, which can occupy intermediate values. These qubits can operate cooperatively through a strange property called quantum entanglement. Multiply those interactions over billions of switches and you get a computer that is extraordinarily powerful at tackling certain computational challenges that classical computers cannot, such as simulating quantum systems.
Quantum communication opens up multiple areas, including a new realm of cybersecurity. Like in classical computing, classical information signals are transmitted using bits. These bits can be intercepted and copied by hackers without leaving a trace. Quantum communication uses qubits of information that are transmitted in a fragile intermediate quantum state. If these qubits are intercepted, the fragile state is broken, leaving traces of eavesdropping.
Quantum sensors exploit the fragility of the quantum state to make ultra-sensitive measurements. One example of quantum sensing is the use of entangled light to improve image and sensor resolution. The sensors are expected to yield extremely precise measurements of both optical and non-optical systems.
Quantum foundational science lays the groundwork for the utilization of quantum technologies. Basic research—both experimental and theoretical—is necessary to provide a better understanding of how these technologies can be developed, used, and improved. Materials science plays an integral role in the development of quantum technologies.
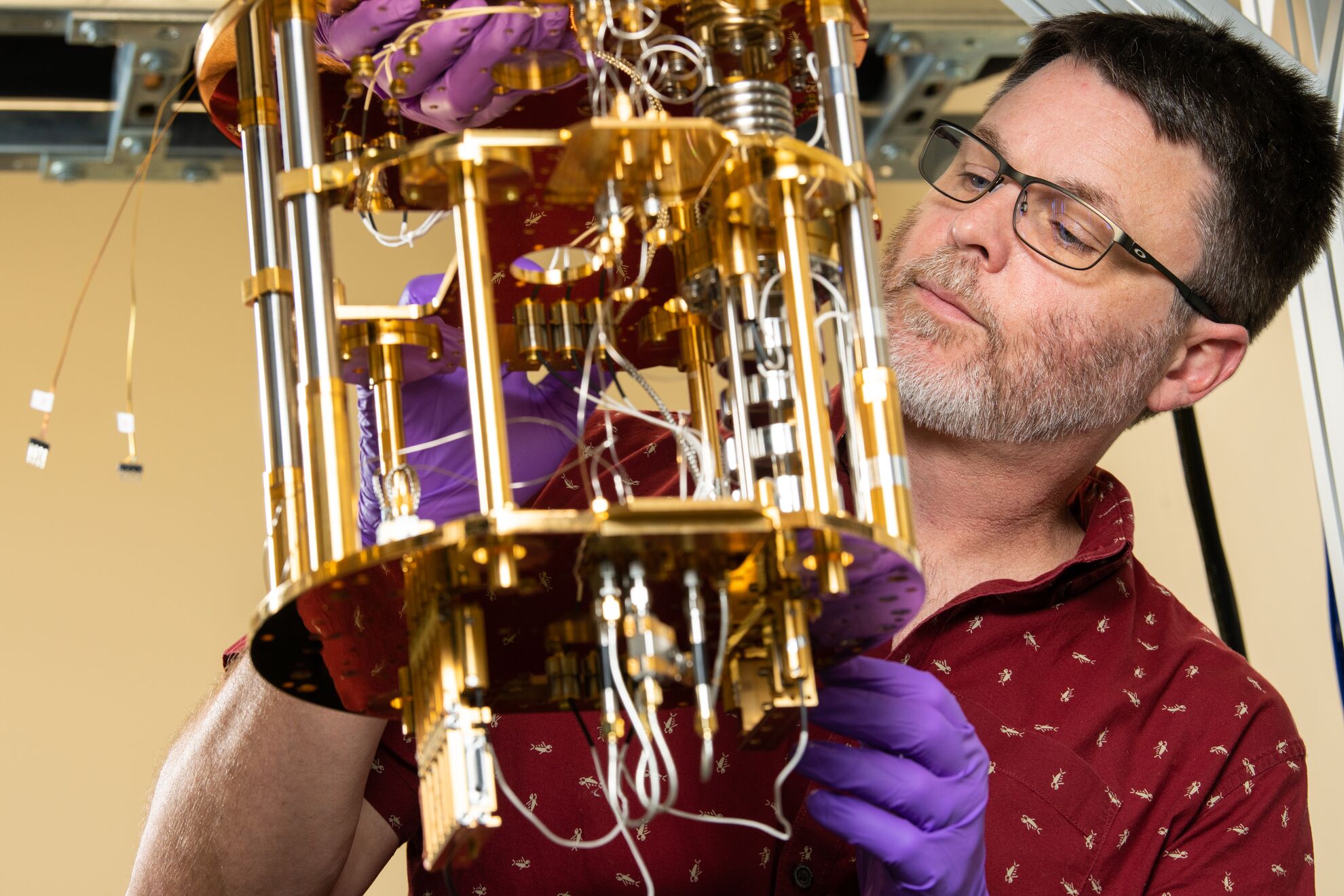
Development of quantum information science
The series of discoveries that make quantum computing possible began a century ago with quantum mechanics. Many scientists working independently provided the theoretical foundation for quantum mechanics drawing from multiple disciplines.
In the 1960s, quantum information theory first appeared as a formulation for optical communication using quantum mechanics.
In 1980, Paul Benioff developed the first theoretical model of a quantum computer. By the late 1980s, a physical model of a computer was developed. Interest in quantum information systems remained relatively stagnant until 1994, when Peter Shor introduced Shor’s algorithm, which allows quantum computers to factor large integers and potentially break many cryptosystems.
The first nuclear magnetic resonance-based quantum computer was demonstrated in the late 1990s with contributions from Los Alamos National Laboratory. Since then, scientists developed and improved upon technologies instrumental in the production of quantum technologies, including two dimensional ion traps, quantum dots, single photon emitters for optical fibers, and single electron qubit memory. Over the decades, the Department of Energy (DOE) supported the advancement of quantum technologies through basic research at many national laboratories and universities.
The National Quantum Initiative Act of 2018 provided the United States a plan for promoting quantum technologies. The National Quantum Initiative is the umbrella under which DOE, the National Institute of Standards and Technology, and the National Science Foundation are collaborating to create a sustainable environment in the United States for quantum research. Though quantum technologies are still in an early stage of development, researchers across the government, industry and academia are collaboratively and independently working to advance them.
Why does quantum information science matter?
QIS brings together quantum mechanics with information and computer science to develop theories, algorithms, and technologies that can allow us to surpass the limits of classical computation. It will lay the foundation for many future technologies and play a large role in our economy and national security.
Quantum computers are especially adept at simultaneously considering large numbers of possible combinations. They are expected to be able to factor large numbers and simulate complex molecular behaviors—although many of these applications are years or decades away.
The high sensitivity and precision of quantum sensors is predicted to impact many areas of science. They may be able to improve the resolution in microscopy, replace current global positioning systems, and detect gravitational waves.
Certain types of quantum communication technologies, such as quantum key distribution (QKD), are already being used. With QKD, encrypted data is transmitted as classical bits, while the decryption key is distributed in a quantum state using qubits. Because of the qubits inherent fragility, the quantum state of some of the qubits will collapse if a hacker intercepts the key. This allows for secure communication.
QIS has been recognized as a critical priority for the United States with the passage of the National Quantum Initiative Act in 2018. The Department of Energy Office of Science plays an integral role in the National Quantum Initiative by supporting the fundamental science that underlies quantum computing, simulation, communication, and the creation of tools and instrumentation used in quantum technology development.
Because QIS is still in the early stages of research and development, it is difficult to predict the exact impact that quantum technologies will have on our lives. Though some quantum technologies have been developed, much more research needs to be done before QIS technologies can become widespread.
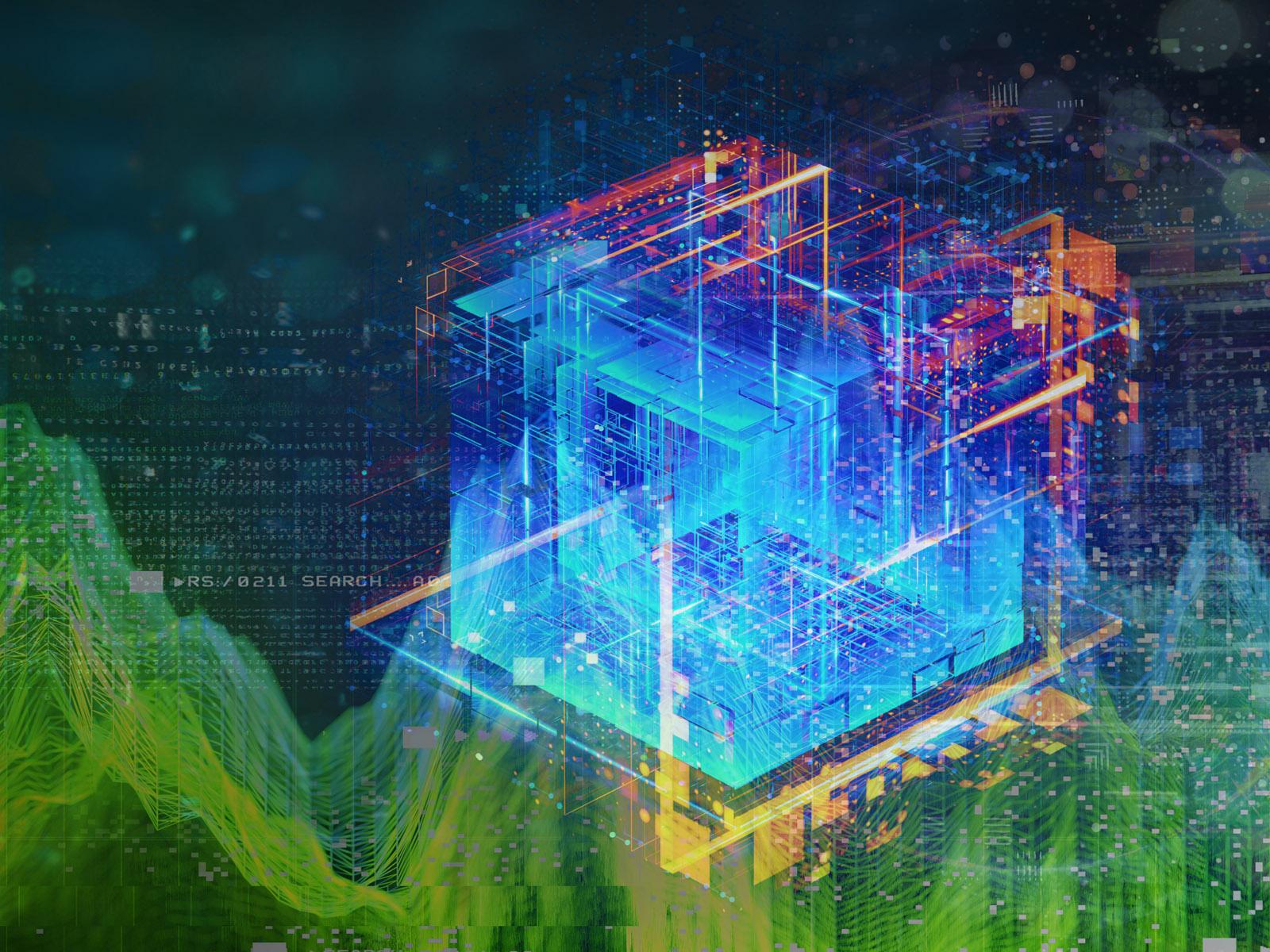
Quantum information sciences at PNNL
Quantum computing is expected to revolutionize the ability for science to solve difficult problems that are beyond the scope of today’s computers. Many of these problems are central to Pacific Northwest National Laboratory (PNNL) core mission areas in chemistry, materials science, and energy resiliency.
Research that advances quantum computing, simulation, communication, and sensing is at the core of PNNL’s QIS strategy. Our approach is one of co-design, where advances in the fundamental understanding of quantum phenomena lead to more robust quantum computing infrastructure, which leads to better problem solving in a cycle of continuous improvement. This expertise has been harnessed by three of the five DOE-sponsored QIS research centers: Q-Next, C2AQ, and QSC.
Materials synthesis science and characterization supports quantum computing with highly tailored, atomically precise materials. PNNL is studying the role of crystallinity as well as chemical and isotopic purity through synthesis, fabrication, and device application. Using microscopy and trace analysis, we are conducting material characterization to relate structure and purity to device performance. Potential uses include new types of qubits and new materials to insulate quantum computers from interference.
PNNL’s strength in physics, our suite of advanced analytical instrumentation, and our Shallow Underground Laboratory are being used to understand quantum computing architectures, which operate at extremely low temperatures. Our expertise in measuring extremely low levels of environmental radiation is being utilized to mitigate environmental disturbances that affect qubit performance. The goal is to advance the development of superconducting quantum computing devices fabricated from well characterized, ultra-precise materials.
Scientists working in the field of quantum chemistry aim to predict the behavior of electrons that orbit an atomic nucleus. At this scale, behavior is described using a large number of parameters—calculations of which that can overwhelm even the most powerful supercomputers. For this reason, quantum chemistry has been identified as a leading application for quantum computing. Additionally, chemical formulations can efficiently be mapped on quantum computing algorithms. PNNL is developing quantum computing algorithms and programming models that demonstrate the power of quantum computing with quantum-accelerated applications. Much of this work is built from the backbone of NWChem computational chemistry code.
PNNL researchers are also working on QIS under the Chemical Dynamics Initiative. Under the initiative research focus, functional materials use case, scientists develop methods and techniques to predict and understand chemical dynamics at the surfaces and interfaces of atomically precise materials, including those used in quantum technologies.
PNNL is also a founding partner in the regional Northwest Quantum Nexus (NQN), a coalition of research and industrial organizations in the Pacific Northwest and neighboring regions with the goal of advancing QIS research and developing a QIS-trained workforce. Microsoft and the University of Washington are its co-founders. Washington State University and IonQ have recently been accepted membership in the NQN, and other universities and commercial entities are expected to follow. The relationships between the University of Washington and Microsoft have found connections in the National Quantum Initiative via the DOE and National Science Foundation programs. The NQN has hosted scientific seminars to engage with the state of Washington on economic and workforce development matters.
Additionally, PNNL is a member of the Quantum Economic Development Consortium (QED-C) created by the National Institute of Standards and Technology. QED-C facilitates the commercialization of quantum technologies by bringing together stakeholders from government, industry, and academia. In 2021, the QED-C established a technology advisory committee for quantum in national security, called Q4NS, in which PNNL helps lead.
